Workshop TTI.5: Mines Paris – PSL at the heart of the challenges linked to the electrification of uses for the ecological transition
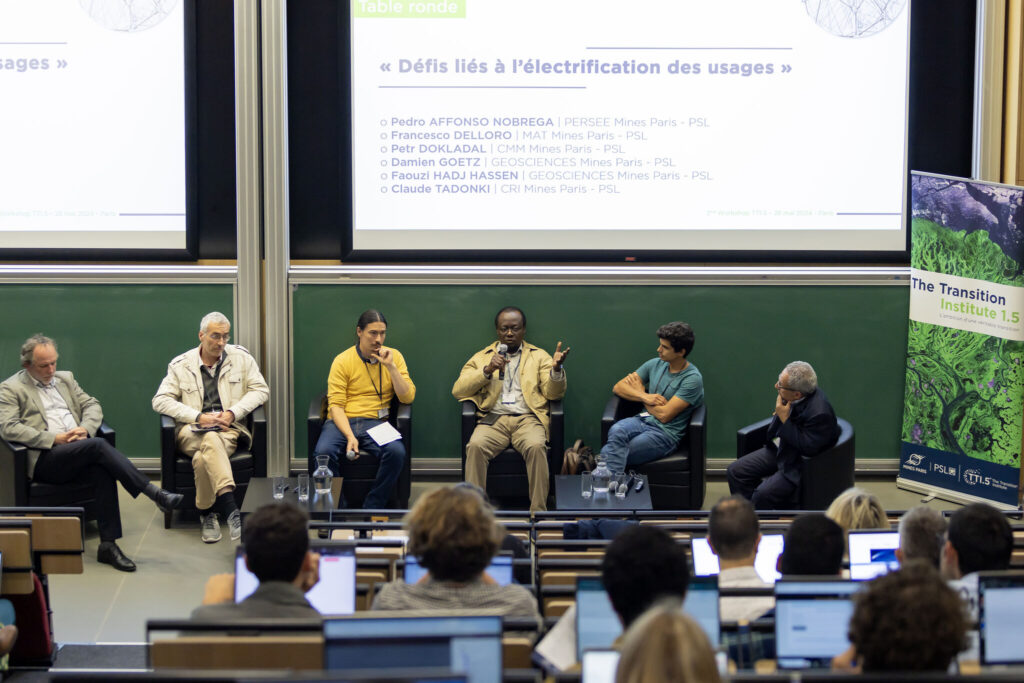
The ambition of this workshop is to give participants the opportunity to present their work, exchange ideas and collaborate in an environment conducive to discussion and reflection. Through these days, The Transition Institute 1.5’s aspiration is to make a significant contribution to the advancement of research and collective understanding of the issues involved in low-carbon transition. These roundtables, structured around TTI.5’s research areas, enable in-depth, collective reflection on environmental debates.
The research theme of this round table, “An electric planet?”, aims to question the relevance of deploying the electric vector, often presented as an unavoidable solution for the energy transition. The aim is to define the technical, social, organizational and political conditions required to implement low-carbon transition trajectories at different scales. By exploring the issues inherent in electrification, researchers at Mines Paris – PSL aim to propose viable, sustainable solutions.
To achieve these objectives, it is essential to focus on the technological and consumption trajectories compatible with decarbonized electrification. This includes an in-depth analysis of the operational challenges for power systems, production technologies, available energy carriers and resources, and contemporary lifestyles. The economic and political levers likely to facilitate the implementation of these trajectories are also at the heart of this reflection.
Damien Goetz, researcher at the Centre de GEOSCIENCES at Mines Paris – PSL, discusses the idea that electrification is often presented as the only alternative that can be mastered on a large scale to meet current climate challenges. However, the availability of this energy varies considerably from one country to the next, sometimes making the supply little green or insufficient. The challenge is therefore to cover all stages in the life of electrical energy, from production to consumption.
Historically, the world economy has relied on oil, but given the need to move away from the oil system and the consideration of a transition to electric power, the central question is now when we will run out of lithium and other essential minerals. This subject, though critical, often remains remote from societal concerns, and involves complex geological and economic dimensions. Over the past 15 years, the number of known sources of lithium has increased sevenfold. Research conducted at Mines Paris – PSL and elsewhere is actively examining the possibility of extracting lithium from regions such as the Allier or the geothermal brines of Alsace. However, the economic dimension of lithium mining is just as crucial. Reserves are closely linked to market prices, and proving the profitability of a deposit is costly.
Petr Dokladal, research fellow at the Centre de Morphologie Mathématique (CMM) at Mines Paris – PSL, raised the question of industrial capacity to meet the needs of the energy transition. According to Damien Goetz, we can no longer rely on the expansion of oil extraction to meet energy needs. Five years ago, the International Energy Agency (IEA), when planning the ecological transition, did not take into account these new metal requirements, which are crucial to its implementation today. The IEA now recognizes that the initial trajectory is unfeasible: it’s too late to launch new projects within the required timeframe. The transition will therefore take place either by adopting energy sobriety, or by advancing at the rate at which the necessary metals can be produced.
Faced with this reality, another question arises: aren’t we just replacing one evil with another? Damien Goetz admits that any energy transition involves pollution. The environmental impacts are undeniable, particularly when it comes to water consumption. For example, in South America, particularly in Chile, almost all copper mining operations use desalinated seawater, pumped from the coast to mining sites in the mountains, in the absence of authorizations to use local water. This geographical disparity leads to unequal treatment in terms of environmental impact.
The workshop also addressed the challenges of underground energy storage. Faouzi Hadj Hassen, teacher-researcher at the GEOSCIENCES Centre at Mines Paris – PSL, emphasized the importance of underground spaces for the massive storage of hydrocarbons in gaseous or liquid form. In fact, existing natural reservoirs, notably exploited hydrocarbon deposits, make up 80% of natural porous environments suitable for this type of storage. For hydrogen, for example, aquifers account for 13% of storage capacity. Using these reservoirs, it is possible to create massive, large-scale storage by expelling water through gas compression.
A second storage method consists of creating artificial cavities, particularly in saline formations, sometimes up to 1500m deep. These cavities are relatively easy to create, by injecting fresh water to dissolve the salt, although the extracted brine requires the intervention of chemists for its use in industry. Thanks to its low permeability and inertia, salt works like a gas canister, withstanding high pressures and enabling immediate reactivity in times of high demand, which is ideal for the energy transition.
Another variant of artificial cavities uses explosives to mine and create impermeable linings, a complex task that limits the number of projects of this type. However, regions with no salt formations are still exploring this method. Historically, underground storage has been for hydrocarbons, and recently projects have turned to compressed air to store renewable energy. Although the yields of this method are low, it can meet peaks in energy demand, underlining the importance of diversifying storage solutions to support the energy transition, while responding to specific geographical and technical particularities.
The use of fuel cell cars remains a major technological and economic challenge, as Pedro Affonso Nobrega, research fellow at the Centre Procédés, Énergies renouvelables et Systèmes énergétiques (PERSEE) at Mines Paris – PSL, explains. Fuel cells, while promising for their efficiency and speed of response, are far from ready for mass adoption. Indeed, these devices, based on rare-earth ceramics, present difficulties in terms of mechanical and thermal robustness, limiting their durability.
On the economic and practical front, several challenges remain to improve the customer experience. For example, although the refuelling time for a fuel cell vehicle is shorter (between 6 and 10 minutes) than for conventional electric vehicles, it is still longer than for combustion-powered cars. In addition, fuel cell cold-start problems, although not blocking, still require improvement to ensure optimum reliability. For a customer who has invested a considerable sum in an electric vehicle, these aspects need to be resolved to make this technology more attractive and viable.
Claude Tadonki, researcher at the Centre de Recherche en Informatique (CRI) at Mines Paris – PSL, highlighted the energy impact of supercomputers. High-Performance Computing (HPC), defined as the ability to perform calculations quickly and efficiently, is a technological feat that leads to a worrying increase in energy consumption. The intensive use of these supercomputers, essential for various everyday applications, exacerbates this problem. For example, ChatGPT consumes the equivalent energy of 17,000 homes (in the USA) every day, highlighting the power-hungry nature of these systems.
In France, as in most developed countries, supercomputer energy consumption has increased significantly, leading to an imminent shortfall in electricity requirements. The associated costs are exorbitant: ChatGPT’s supercomputer costs around $7 million a year in electricity alone. What’s more, only 3% of a supercomputer’s energy is used for computation, the rest being dedicated to cooling the infrastructure, underlining the glaring energy inefficiency.
The massive use of cutting-edge technologies for computer processing also places a heavy burden on natural resources, particularly those involved in the production of the necessary electricity, resulting in an additional cost in terms of carbon footprint. To mitigate these impacts, it is vital to turn to innovative solutions. It is becoming necessary to design programs that prioritize efficiency over speed, and to implement less energy-intensive cooling techniques such as the use of cold lake water. The European Green Deal also proposes strict standards for data centers, aimed at building less polluting infrastructures. These initiatives are essential to controlling energy consumption and reducing the environmental impact of high-performance computing, a technology that is both indispensable and demanding.
On May 28, 2024, the Mines Paris – PSL campus hosted the second edition of the TTI.5 Workshop, organized by The Transition Institute 1.5 (TTI.5). This...